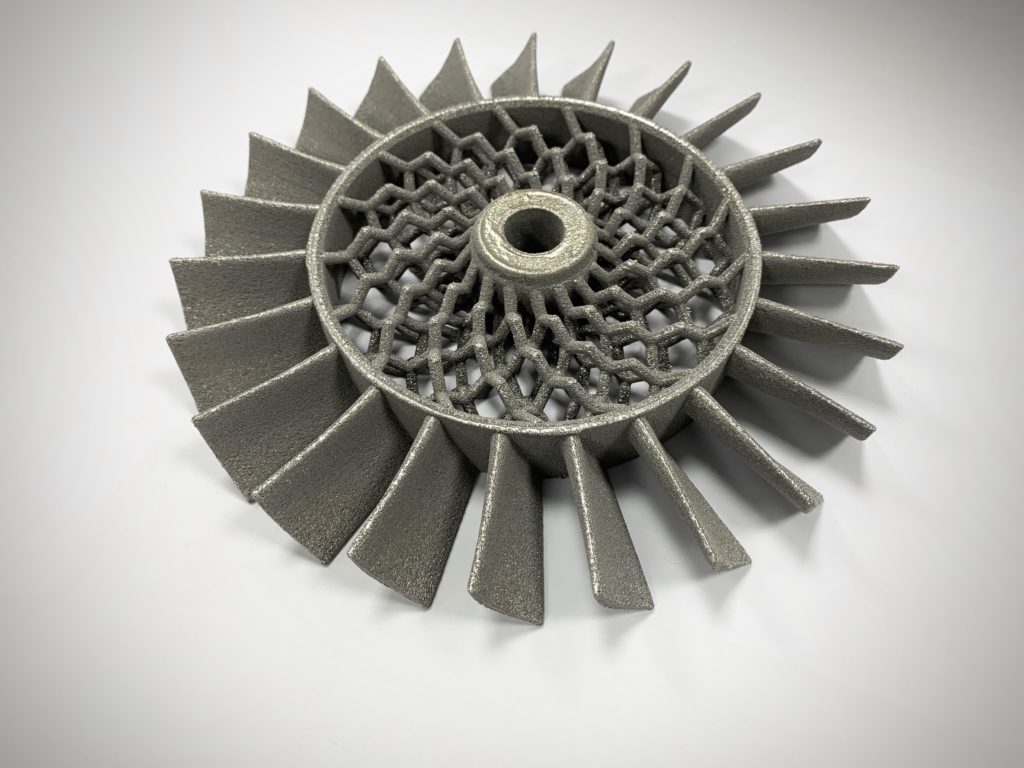
Applications using metal additive manufacturing (AM) processes continue to increase year on year with considerable R&D activities across all key industrial sectors. The generic term “metal AM” provides an umbrella for a number of specific but very different metal processes that produce parts additively. These different processes offer different advantages that can be utilised to suit specific applications, but until now — and without exception — they also come with mutliple limitations that require compromises to be made (or found). This article will consider the different metal AM process options available to industry, with a primary focus on the powder bed fusion (PBF) approach and highlight a new development — the NeuBeam process — that greatly reduces the requirement for compromise.
Introduction
Across many industry sectors, there is a continued drive to adopt metal additive manufacturing (AM) for production applications as an alternative to traditional fabrication processes. A key driver in this respect is the ability to produce geometrically complex and innovative products that are laborious, time consuming, restrictive, and cost prohibitive with conventional manufacturing processes.
New applications continue to emerge as manufacturers find ways to apply metal AM and harness its advantages. However, the rate of development is not as fast as many predicted even just a few years ago, because AM — particularly with metal materials — comes with a number of limitations of its own. Identifying applications that minimise these limitations is, unsurprisingly, not that easy.
At Wayland Additive, we have been working on the development of an entirely new powder bed fusion (PBF) process for metal AM that minimises the existing limitations that current users have to work around. When considering the existing two PBF technologies, NeuBeam offers a new way, a 3rd way.
Metal AM Processes
Of the seven different process classifications for AM, there are three that are well established for metal.
- Powder bed fusion (PBF): utilizing a high power heat source — either a laser or an electron beam (eBeam) — to melt metal powders together, a layer at a time within a powder bed.
- Direct metal deposition (DMD): molten metal powder / wire is deposited onto an existing substrate in thin layers.
- Binder jetting: a powder bed process that uses a base metal powder material and a liquid binder material that is deposited a layer at a time to bind the powder.
This article is primarily focused on the most established metal AM processes for production applications — PBF.
Laser vs eBeam for PBF
PBF is arguably the most widely adopted AM process for structural production applications. This is partly down to process capabilities and partly due to the maturity of the technology. To date there has been a clear two-way choice with PBF, users either having the option to use laser-based PBF systems or eBeam PBF technology. Both of these metal PBF processes have been around since the 1990’s.
The most obvious difference between the two is the heat source used to melt the metal powder, indicated in the process names. Laser PBF typically utilizes a high powered 200-400 watt Yb-fiber optic laser (or series of lasers), while traditional EBM uses an eBeam in the region of 3–6 kW and a magnetic field to direct the beam. The very nature of the eBeam requires that the process occurs under vacuum, because any gas molecules in the chamber would adversely affect the beam, and the vacuum conditions also ensure safe operation. In contrast, the operating conditions for laser PBF platforms require inert gas, typically nitrogen or argon.
One trade-off between the two technologies comes down to precision versus speed. While laser PBF has traditionally held the advantage in terms of fidelity and surface finish (due to the precise nature of the laser(s)), eBeam PBF gains significant advantage in terms of speed and productivity as a result of the more efficient way that electrons transmit energy to the powder bed, and through-thickness heating of the entire layer. In addition, eBeam can process multiple meltpools simultaneously, further contributing to increased productivity.
Specific Benefits of Laser PBF
Laser PBF is the oldest industrial metal AM process and as stated utilizes precise, high-power lasers to melt powdered metals and alloys to form accurate, complex and fully-functional metal parts. It is wide recognized as a stable process, that can process a relatively broad range of metal materials. The process can process very fine powders and therefore can achieve fine layer thicknesses (typically 20–40 µm, compared with 50–90 µm for EBM) and as a result produces parts with finer details and smoother surface finishes.
Specific Benefits of eBeam PBF
The eBeam PBF process is recognised as exhibiting favourable energy transfer physics and
is characterised by superior metallurgy, with substantially fewer issues concerning residual stress than laser PBF. This is because eBeam PBF operates at higher processing temperatures that minimize residual stresses and thus distortion and failed builds due to cracking / mechanical failure. In addition, eBeam PBF permits good material properties, producing fully dense parts, and without contamination from highly oxidized particles seen in laser PBF [1].
However ….
It is no secret in industry that existing Laser and eBeam PBF systems come with limitations, some of them prohibitive. To be clear (and fair) both have — rightly — proved valuable for certain industrial applications. Although invariably this also necessitates compromise(s) to overcome their limitations, and sometimes, these compromises can negate the actual benefits of using AM in the first place.
With Laser PBF, the resulting internal residual stresses that the process generates requires extensive structural supports to prevent the parts distorting or cracking during the print process. Sometimes these supports require as much material to manufacture as the parts themselves. Removing these supports post-build typically requires the use of wire EDM and machining operations, which are expensive and time consuming. The parts typically also require stress-relieving in a furnace post-build – another operation, more cost, and another piece of equipment to maintain and run.
Laser PBF parts tend to be manufactured on support plates that are manufactured from a similar material to the printed part (Titanium parts are printed on Titanium plates etc). This is to ensure that the support material properly welds to the plate. As build volume size increases, the thickness of the plate also tends to increase, with very large parts requiring plates which may be several inches thick. For expensive materials such as Titanium this can be a considerable cost, especially if plates are lost to large distortion due to residual stresses. Notably, the eBeam PBF process can use thin plates made of cheaper materials [2], and thus does not require wire EDM to remove parts, and has many fewer supports.
With Laser PBF, the process also suffers from the production of highly-oxidised particles (“spatter”) being ejected from the melt pool [3]. Typically a cross-flow of Argon gas is used to carry away these highly-oxidised particles to a filter, but still a minority of these particles land in the powder bed and cause localised contamination, which can affect mechanical properties of the parts [4]. This cross-flowing gas contaminated with spatter is apparent as a plume of smoke emanating from the melt pool and carried in the downstream direction. When processing using multiple lasers, care has to be taken to ensure that one laser is not processing downstream of another as it can be obscured by the smoke. As the size of powder beds increases, it becomes increasingly difficult to maintain an even laminar flow of gas across the powder bed, and this tends to limit the feasible size of parts produced by laser PBF machines.
Similarly, the traditional EBM process faces its own challenges and constraints. First, users are often frustrated by the instability of the process. This instability is caused by charge accumulation within the build chamber, which can result in powder scattering or a so-called “smoke event” that distorts the current layer of the build and therefore compromises the entire build. To avoid this, the process has to be operated in a very specific way, and has a steep learning curve. Particularly, it is critical to maintain the temperature of the powder bed between strict limits, which is required to cause the powder bed to sinter so that it isn’t disturbed by powder charging [5]. This unreliability means that eBeam PBF has typically been considered less favourable than laser-based PBF.
The eBeam PBF process does however lead to downstream complexities that often negate the overall advantages of it. For example, the process entombs the parts in a sintered powder “cake”, which makes part removal and post-processing very difficult, time-consuming and expensive. The challenge of removing unused powder from the parts also imposes geometry limitations on parts, for example with enclosed regions such as cooling channels on a turbine blade.
A 3rd Way — NeuBeam®
NeuBeam is an entirely new PBF process that is truly ground-breaking in nature and which offers the best of both laser and eBeam PBF without the compromises, and opens up greater potential for more industrial applications. This new process is an eBeam PBF process that effectively neutralises the charge accumulation generated by the electron beam. This offers greater flexibility than laser PBF while overcoming the stability issues of eBeam PBF. In addition, this means that the NeuBeam process enables metallurgical requirements to be tailored to application requirements rather to maintain the print process within the narrow bounds permitted by the process. These process capabilities, along with the greatly improved ease of process development, also opens up the use of a much wider range of metal materials.
Despite a couple of commonalities between EBM and NeuBeam® — namely that they are both PBF processes and they use an electron beam as the heat source to melt the metal powder — it is important to understand that EBM and NeuBeam are fundamentally different. Unlike the traditional eBeam PBF process, the charging issues that make EBM so unstable have been fully neutralized with NeuBeam using core physics principles developed in the demanding semi-conductor industry. Moreover, NeuBeam is a hot part process rather than a hot bed process. This efficiently creates parts that are free of residual stresses because the high temperatures are only applied to the part and not the bed, ensuring free-flowing powder post-build (no sinter cake) and stress free parts with reduced energy consumption.
Furthermore, the process overcomes many of the limitations for manufacturing large components – no residual thermal stresses, no gas cross-flow, and a much simplified powder removal process than existing eBeam systems.
The NeuBeam process is capable of producing fully dense parts in a wide range of materials, many of which are not compatible with traditional eBeam or laser PBF processes such as refractory metals and highly reflective alloys. As a result, the NeuBeam process can demonstrate vastly improved metallurgy, without many of the compromises that existing metal AM processes necessitate.
NeuBeam also offers significant advantages over other technologies with built-in real-time in-process monitoring, allowing for rapid material development or tuning of microstructures by adapting the solidification during manufacture. With NeuBeam the process temperature is not constrained by sintering the powder bed, allowing the process temperature to be optimized to the material microstructure and/or the application.
These unprecedented levels of in-process monitoring are achieved through a combination of advanced technologies, including structured light scanning, electron imaging and high speed infra-red cameras. Each of these different monitoring approaches are calibrated to the same reference points in the machine, with managed adjustments, to ensure optimum results and output. Being a true thermal process, everything in the build chamber is able to be monitored, and it is possible to see the true temperature of the whole powder bed in-process as a build takes place. The thermal history of the material being processed can also be seen, as can the topography of the surface so that defects can be detected — and reported — as they occur.
ABOUT WAYLAND ADDITIVE
NeuBeam technology has been developed in-house from the ground up by a team of physicists that have worked for many decades with electron beam technology and industrial systems in the semi-conductor industry. The science, combined with extensive expertise and experience has allowed Wayland Additive to develop a very capable and reliable system rather than imitating existing systems or adapting off-the-shelf components and re-purposing them.
REFERENCES
[1] C. L. A. Leung, S. Marussi, M. Towrie, R. C. Atwood, P. J. Withers, and P. D. Lee, “The effect of powder oxidation on defect formation in laser additive manufacturing,” Acta Mater., vol. 166, pp. 294–305, 2019.
[2] S. S. Al-Bermani, M. L. Blackmore, W. Zhang, and I. Todd, “The origin of microstructural diversity, texture, and mechanical properties in electron beam melted Ti-6Al-4V,” Metall. Mater. Trans. A Phys. Metall. Mater. Sci., vol. 41, no. 13, pp. 3422–3434, 2010.
[3] P. Bidare, I. Bitharas, R. M. Ward, M. M. Attallah, and A. J. Moore, “Fluid and particle dynamics in laser powder bed fusion,” Acta Mater., vol. 142, pp. 107–120, 2018.
[4] M. Velasco-Castro, E. Hernández-Nava, I. A. Figueroa, I. Todd, and R. Goodall, “The effect of oxygen pickup during selective laser melting on the microstructure and mechanical properties of Ti–6Al–4V lattices,” Heliyon, vol. 5, no. 12, Dec. 2019.
[5] Z. C. Cordero, H. M. Meyer, P. Nandwana, and R. R. Dehoff, “Powder bed charging during electron-beam additive manufacturing,” Acta Mater., vol. 124, pp. 437–445, 2017.
More Stories
Sensor Fusion and the Next Generation of Autonomous Driving Systems
Unique Experiences in Miami: What to Do Off the Beaten Path
The Factors That Impact the Outcome of a Car Accident Claim